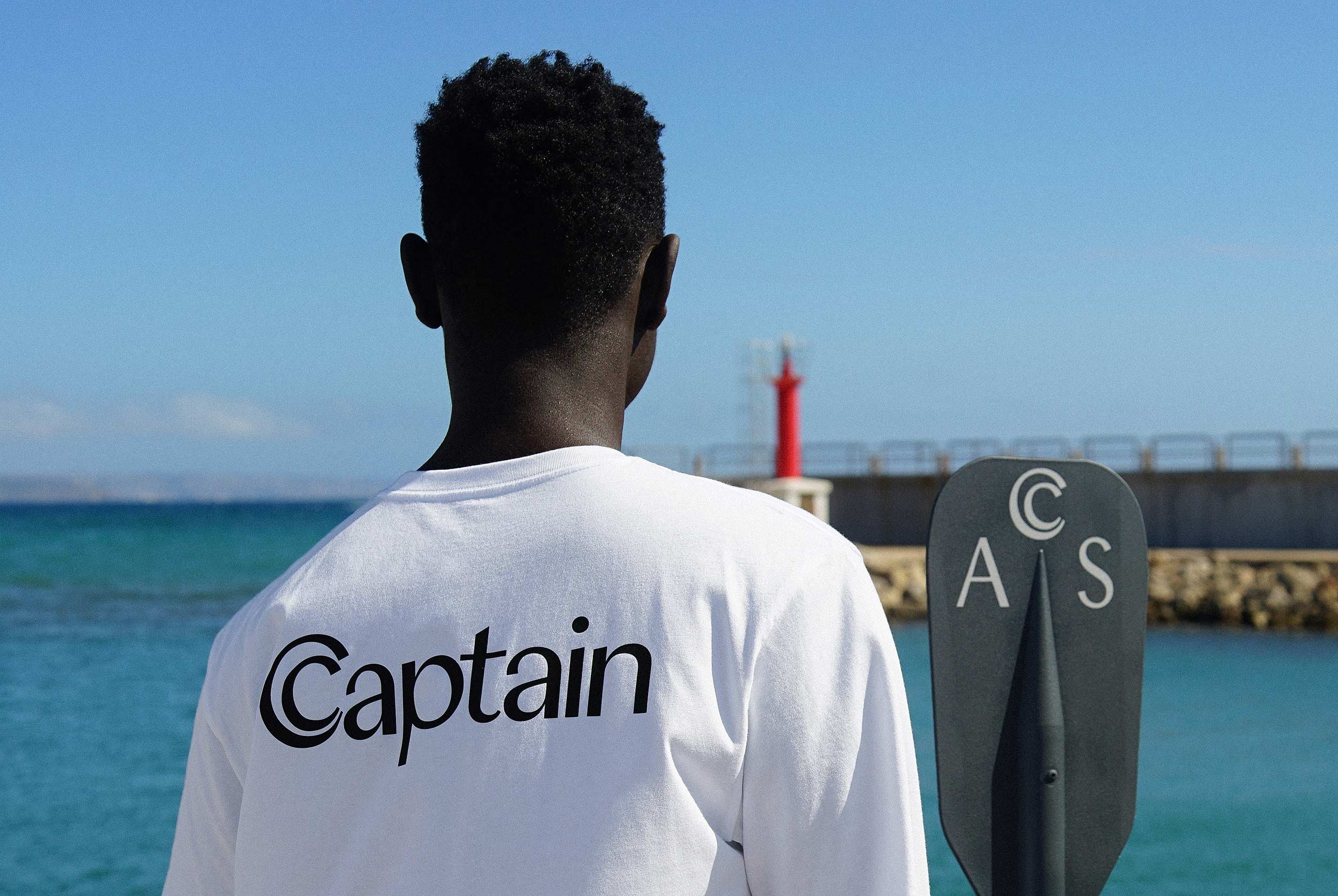
-
Atlantic Bold735 W. Lyme Street
North Miami Beach, FL 33160 -
Atlantic SemiBold735 W. Lyme Street
North Miami Beach, FL 33160 -
Atlantic Medium735 W. Lyme Street
North Miami Beach, FL 33160 -
Atlantic Regular735 W. Lyme Street
North Miami Beach, FL 33160 -
Atlantic Light735 W. Lyme Street
North Miami Beach, FL 33160 -
Atlantic UltraLight735 W. Lyme Street
North Miami Beach, FL 33160 -
Atlantic Thin735 W. Lyme Street
North Miami Beach, FL 33160
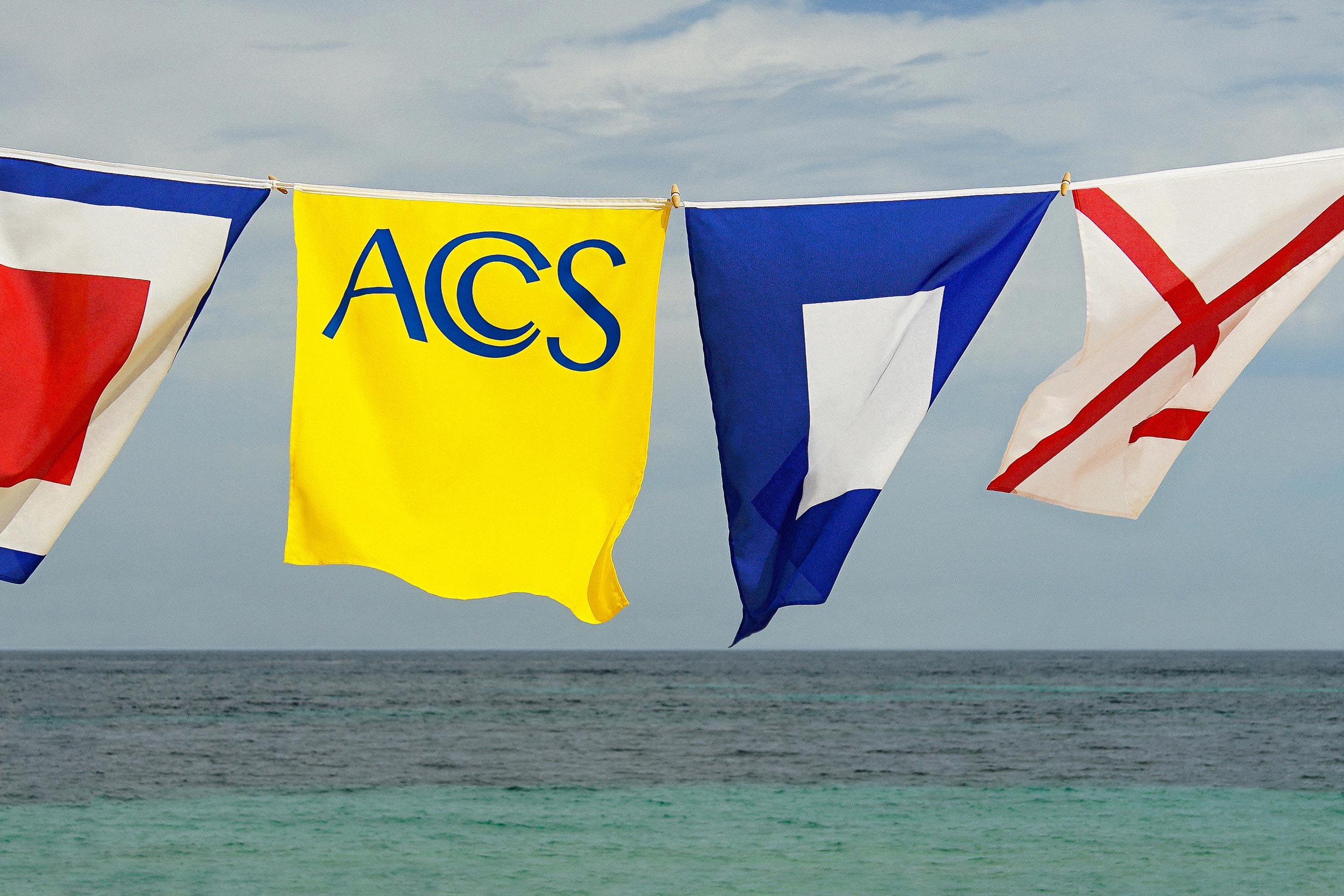
Journal of Geophysical Research
Coordinates:
0°N 25°W
106,460,000 km2
Nautic Area
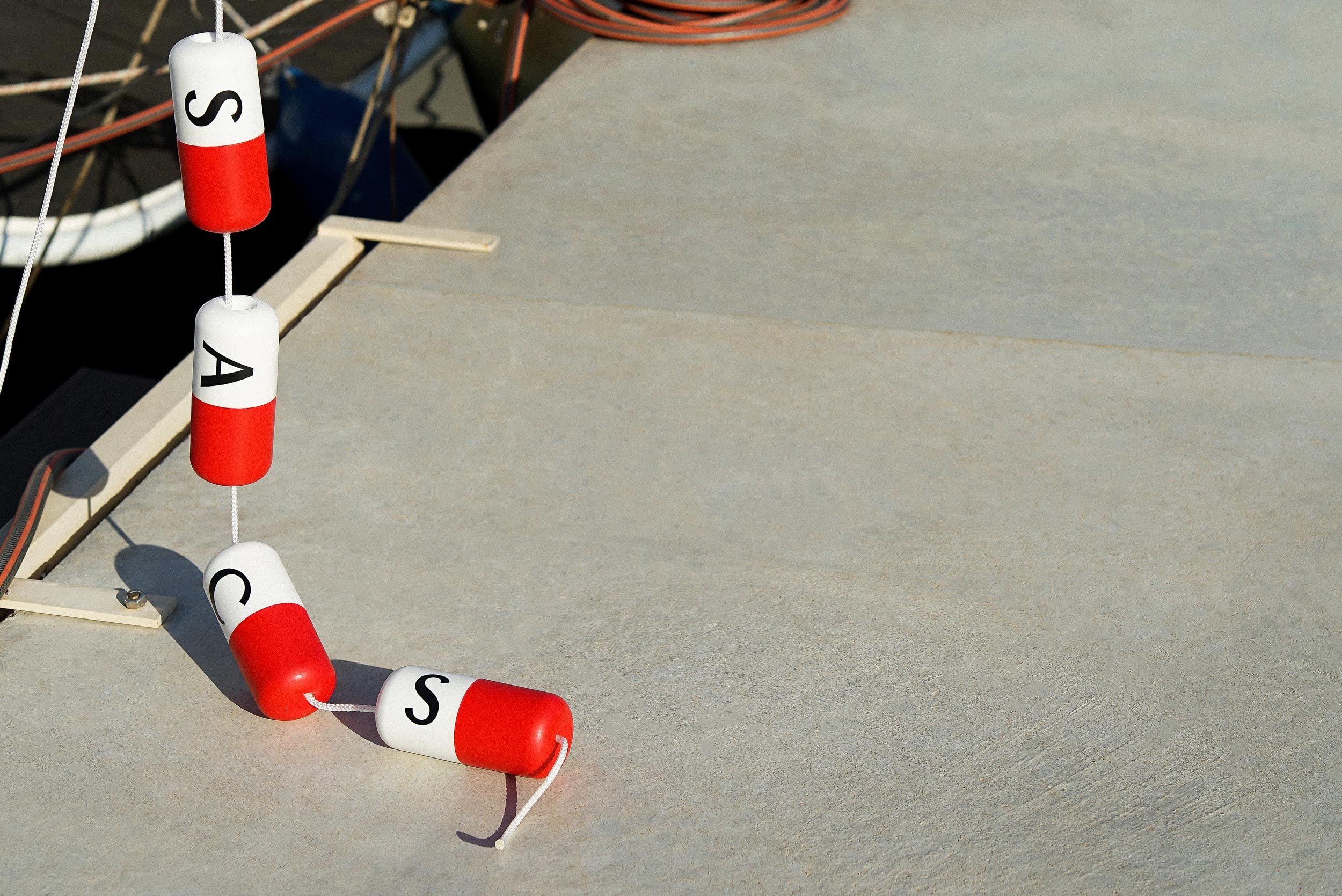
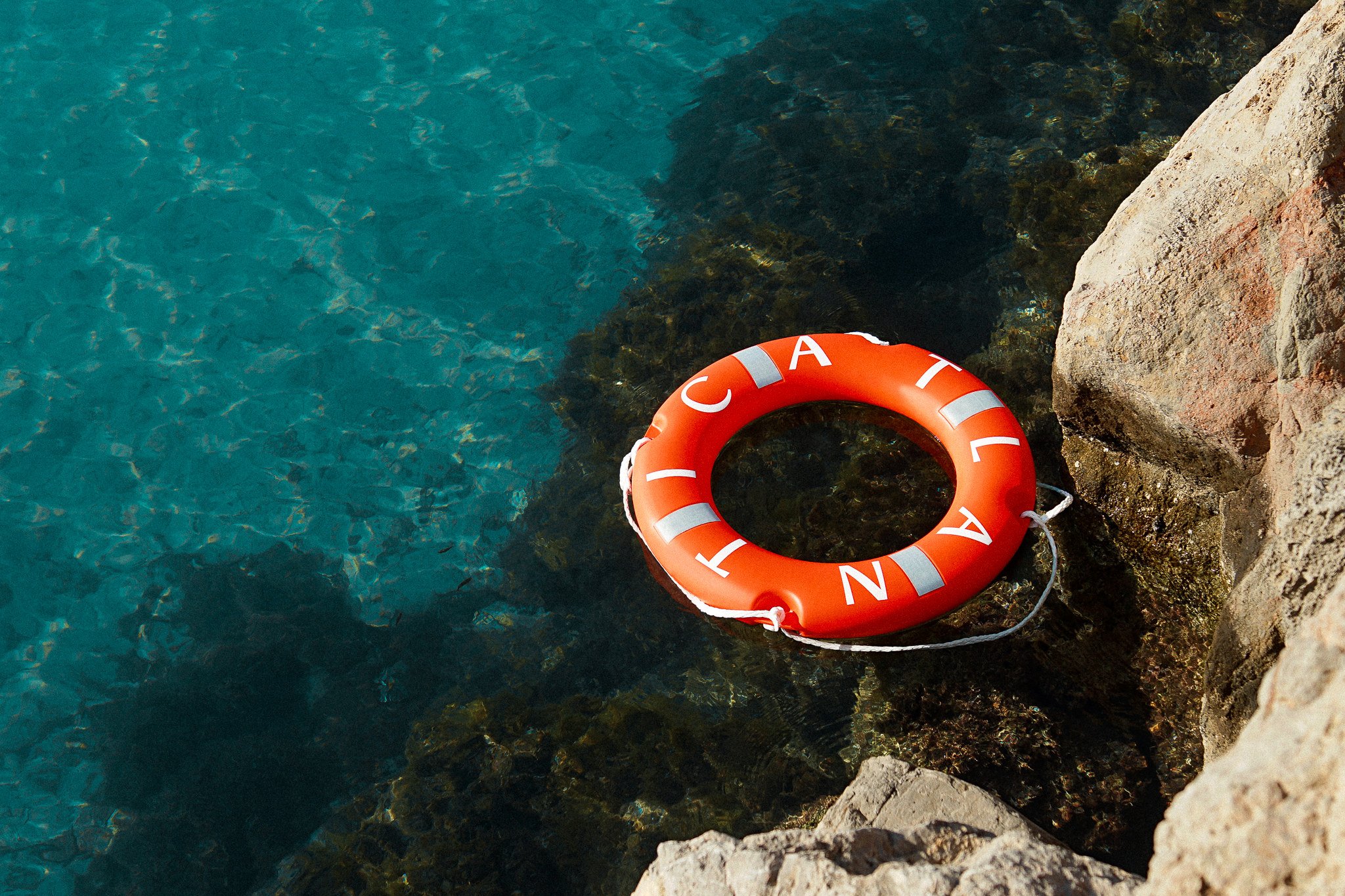
Character overview
Uppercase (26 Glyphs)
-
0041A
-
0042B
-
0043C
-
0044D
-
0045E
-
0046F
-
0047G
-
0048H
-
0049I
-
004AJ
-
004BK
-
004CL
-
004DM
-
004EN
-
004FO
-
0050P
-
0051Q
-
0052R
-
0053S
-
0054T
-
0055U
-
0056V
-
0057W
-
0058X
-
0059Y
-
005AZ
Lowercase (26 Glyphs)
-
0061a
-
0062b
-
0063c
-
0064d
-
0065e
-
0066f
-
0067g
-
0068h
-
0069i
-
006Aj
-
006Bk
-
006Cl
-
006Dm
-
006En
-
006Fo
-
0070p
-
0071q
-
0072r
-
0073s
-
0074t
-
0075u
-
0076v
-
0077w
-
0078x
-
0079y
-
007Az
Uppercase Accents (162 Glyphs)
-
00C1Á
-
0102Ă
-
00C2Â
-
00C4Ä
-
00C0À
-
0100Ā
-
0104Ą
-
00C5Å
-
00C3Ã
-
01FAǺ
-
01CDǍ
-
0200Ȁ
-
0226Ȧ
-
1EA0Ạ
-
0202Ȃ
-
00C6Æ
-
01FCǼ
-
01E2Ǣ
-
1E02Ḃ
-
0106Ć
-
010CČ
-
00C7Ç
-
0108Ĉ
-
010AĊ
-
010EĎ
-
0110Đ
-
00D0Ð
-
1E10Ḑ
-
1E0AḊ
-
1E0CḌ
-
1E0EḎ
-
01C4DŽ
-
01C5Dž
-
00C9É
-
0114Ĕ
-
011AĚ
-
00CAÊ
-
00CBË
-
0116Ė
-
00C8È
-
0112Ē
-
0118Ę
-
0204Ȅ
-
1EB8Ẹ
-
1EBCẼ
-
1E1EḞ
-
011EĞ
-
011CĜ
-
0122Ģ
-
0120Ġ
-
01E6Ǧ
-
1E20Ḡ
-
0126Ħ
-
0124Ĥ
-
1E2AḪ
-
021EȞ
-
1E22Ḣ
-
1E24Ḥ
-
00CDÍ
-
012CĬ
-
00CEÎ
-
00CFÏ
-
0130İ
-
00CCÌ
-
012AĪ
-
012EĮ
-
0128Ĩ
-
01CFǏ
-
0208Ȉ
-
1ECAỊ
-
0197Ɨ
-
0132IJ
-
0134Ĵ
-
0136Ķ
-
01E8Ǩ
-
0139Ĺ
-
013DĽ
-
013BĻ
-
013FĿ
-
1E36Ḷ
-
0141Ł
-
1E3EḾ
-
1E40Ṁ
-
1E42Ṃ
-
0143Ń
-
0147Ň
-
0145Ņ
-
00D1Ñ
-
1E44Ṅ
-
1E46Ṇ
-
01F8Ǹ
-
1E48Ṉ
-
019DƝ
-
014AŊ
-
00D3Ó
-
014EŎ
-
00D4Ô
-
00D6Ö
-
00D2Ò
-
0150Ő
-
014CŌ
-
00D5Õ
-
01D1Ǒ
-
020CȌ
-
1ECCỌ
-
022EȮ
-
01EAǪ
-
00D8Ø
-
01FEǾ
-
0152Œ
-
1E56Ṗ
-
00DEÞ
-
0154Ŕ
-
0158Ř
-
0156Ŗ
-
0210Ȑ
-
1E5AṚ
-
1E5CṜ
-
1E5EṞ
-
015AŚ
-
0160Š
-
015CŜ
-
015EŞ
-
0218Ș
-
1E60Ṡ
-
1E62Ṣ
-
00DFß
-
0166Ŧ
-
0164Ť
-
0162Ţ
-
021AȚ
-
1E6AṪ
-
1E6CṬ
-
00DAÚ
-
016CŬ
-
00DBÛ
-
00DCÜ
-
0170Ű
-
00D9Ù
-
016AŪ
-
0172Ų
-
016EŮ
-
0168Ũ
-
01D3Ǔ
-
0214Ȕ
-
1EE4Ụ
-
0244Ʉ
-
1E7CṼ
-
1E82Ẃ
-
0174Ŵ
-
1E84Ẅ
-
1E80Ẁ
-
00DDÝ
-
0176Ŷ
-
0178Ÿ
-
1EF2Ỳ
-
0232Ȳ
-
1EF8Ỹ
-
0179Ź
-
017DŽ
-
017BŻ
-
1E92Ẓ
Lowercase Accents (167 Glyphs)
-
00E1á
-
0103ă
-
00E2â
-
00E4ä
-
00E0à
-
0101ā
-
0105ą
-
00E5å
-
00E3ã
-
01FBǻ
-
01CEǎ
-
0201ȁ
-
0227ȧ
-
1EA1ạ
-
0203ȃ
-
00E6æ
-
01FDǽ
-
01E3ǣ
-
1E03ḃ
-
0107ć
-
010Dč
-
00E7ç
-
0109ĉ
-
010Bċ
-
010Fď
-
1E11ḑ
-
1E0Bḋ
-
1E0Dḍ
-
1E0Fḏ
-
0111đ
-
01C6dž
-
00E9é
-
0115ĕ
-
011Bě
-
00EAê
-
00EBë
-
0117ė
-
00E8è
-
0113ē
-
1EBDẽ
-
0119ę
-
0205ȅ
-
1EB9ẹ
-
0258ɘ
-
0259ə
-
01DDǝ
-
1E1Fḟ
-
011Fğ
-
011Dĝ
-
0123ģ
-
0121ġ
-
01E7ǧ
-
1E21ḡ
-
0127ħ
-
0125ĥ
-
1E2Bḫ
-
021Fȟ
-
1E23ḣ
-
1E25ḥ
-
00EDí
-
012Dĭ
-
00EEî
-
00EFï
-
00ECì
-
012Bī
-
012Fį
-
0129ĩ
-
0131ı
-
01D0ǐ
-
0209ȉ
-
1ECBị
-
0268ɨ
-
0133ij
-
0135ĵ
-
0237ȷ
-
0137ķ
-
01E9ǩ
-
03BAκ
-
013Aĺ
-
013Eľ
-
013Cļ
-
0140ŀ
-
1E37ḷ
-
0142ł
-
1E3Fḿ
-
1E41ṁ
-
1E43ṃ
-
0144ń
-
0148ň
-
0149ʼn
-
0146ņ
-
00F1ñ
-
1E45ṅ
-
1E47ṇ
-
01F9ǹ
-
1E49ṉ
-
0272ɲ
-
014Bŋ
-
00F3ó
-
014Fŏ
-
00F4ô
-
00F6ö
-
00F2ò
-
0151ő
-
014Dō
-
00F5õ
-
01D2ǒ
-
020Dȍ
-
1ECDọ
-
022Fȯ
-
01EBǫ
-
00F8ø
-
01FFǿ
-
0153œ
-
1E57ṗ
-
00FEþ
-
0155ŕ
-
0159ř
-
0157ŗ
-
0211ȑ
-
1E5Bṛ
-
1E5Dṝ
-
1E5Fṟ
-
015Bś
-
0161š
-
015Dŝ
-
015Fş
-
0219ș
-
1E61ṡ
-
1E63ṣ
-
00DFß
-
017Fſ
-
0167ŧ
-
0165ť
-
0163ţ
-
021Bț
-
1E6Bṫ
-
1E6Dṭ
-
00FAú
-
016Dŭ
-
00FBû
-
00FCü
-
0171ű
-
00F9ù
-
016Bū
-
0173ų
-
016Fů
-
0169ũ
-
01D4ǔ
-
0215ȕ
-
1EE5ụ
-
0289ʉ
-
1E7Dṽ
-
1E83ẃ
-
0175ŵ
-
1E85ẅ
-
1E81ẁ
-
00FDý
-
0177ŷ
-
00FFÿ
-
1EF3ỳ
-
0233ȳ
-
1EF9ỹ
-
017Aź
-
017Ež
-
017Cż
-
1E93ẓ
Lowercase Stylistic Set 01 (19 Glyphs)
-
0061a
-
00E1á
-
0103ă
-
00E2â
-
00E4ä
-
00E0à
-
0101ā
-
0105ą
-
00E5å
-
00E3ã
-
01CEǎ
-
0201ȁ
-
0203ȃ
-
01FBǻ
-
0227ȧ
-
1EA1ạ
-
00E6æ
-
01FDǽ
-
01E3ǣ
Ligatures (11 Glyphs)
-
fb
-
ff
-
ffb
-
ffh
-
ffi
-
ffl
-
fi
-
fj
-
fk
-
fl
-
ij
Figures (10 Glyphs)
-
00300
-
00311
-
00322
-
00333
-
00344
-
00355
-
00366
-
00377
-
00388
-
00399
Tabular Lining Figures (10 Glyphs)
-
00300
-
00311
-
00322
-
00333
-
00344
-
00355
-
00366
-
00377
-
00388
-
00399
Case Sensitive Figures (10 Glyphs)
-
00300
-
00311
-
00322
-
00333
-
00344
-
00355
-
00366
-
00377
-
00388
-
00399
Math (9 Glyphs)
-
002B+
-
2212−
-
00D7×
-
00F7÷
-
003D=
-
2260≠
-
0023#
-
0025%
-
2030‰
Currency (9 Glyphs)
-
00A4¤
-
00A2¢
-
20AC€
-
0024$
-
00A3£
-
20AA₪
-
20BF₿
-
20BD₽
-
00A5¥
Fraction (2 Glyphs)
-
2044⁄
-
00BD½
Punctuation (47 Glyphs)
-
002E.
-
002C,
-
003A:
-
003B;
-
2025‥
-
2026…
-
0021!
-
203C‼
-
003F?
-
00A1¡
-
00BF¿
-
002F/
-
005C\
-
005F_
-
002D-
-
00AD
-
2013–
-
2014—
-
00B7·
-
2022•
-
0028(
-
0029)
-
005B[
-
005D]
-
007B{
-
007D}
-
27E8⟨
-
27E9⟩
-
27E6⟦
-
27E7⟧
-
00AB«
-
00BB»
-
2039‹
-
203A›
-
0022"
-
0027'
-
2033″
-
2032′
-
201E„
-
201C“
-
201D”
-
201A‚
-
2018‘
-
2019’
-
002A*
-
2051⁑
-
2042⁂
Case Sensitive Punctuation (20 Glyphs)
-
2033″
-
2032′
-
002D-
-
00AD
-
2013–
-
2014—
-
0028(
-
0029)
-
005B[
-
005D]
-
007B{
-
007D}
-
27E8⟨
-
27E9⟩
-
27E6⟦
-
27E7⟧
-
00AB«
-
00BB»
-
2039‹
-
203A›
Arrows (18 Glyphs)
-
27F5⟵
-
27F6⟶
-
2190←
-
2192→
-
2194↔
-
2191↑
-
2193↓
-
2195↕
-
2197↗
-
2196↖
-
2198↘
-
2199↙
-
21A9↩
-
21B0↰
-
21B1↱
-
21B2↲
-
21B3↳
-
21C6⇆
Case Sensitive Arrows (12 Glyphs)
-
27F5⟵
-
27F6⟶
-
2191↑
-
2197↗
-
2192→
-
2198↘
-
2193↓
-
2199↙
-
2190←
-
2196↖
-
2194↔
-
2195↕
Other (13 Glyphs)
-
0040@
-
0040@
-
0026&
-
00A7§
-
2116№
-
00B0°
-
2020†
-
2021‡
-
00A9©
-
00AE®
-
00B6¶
-
007C|
-
00A6¦
Charset:
Subset:
Weight:
Charset:
Subset:
Weight:
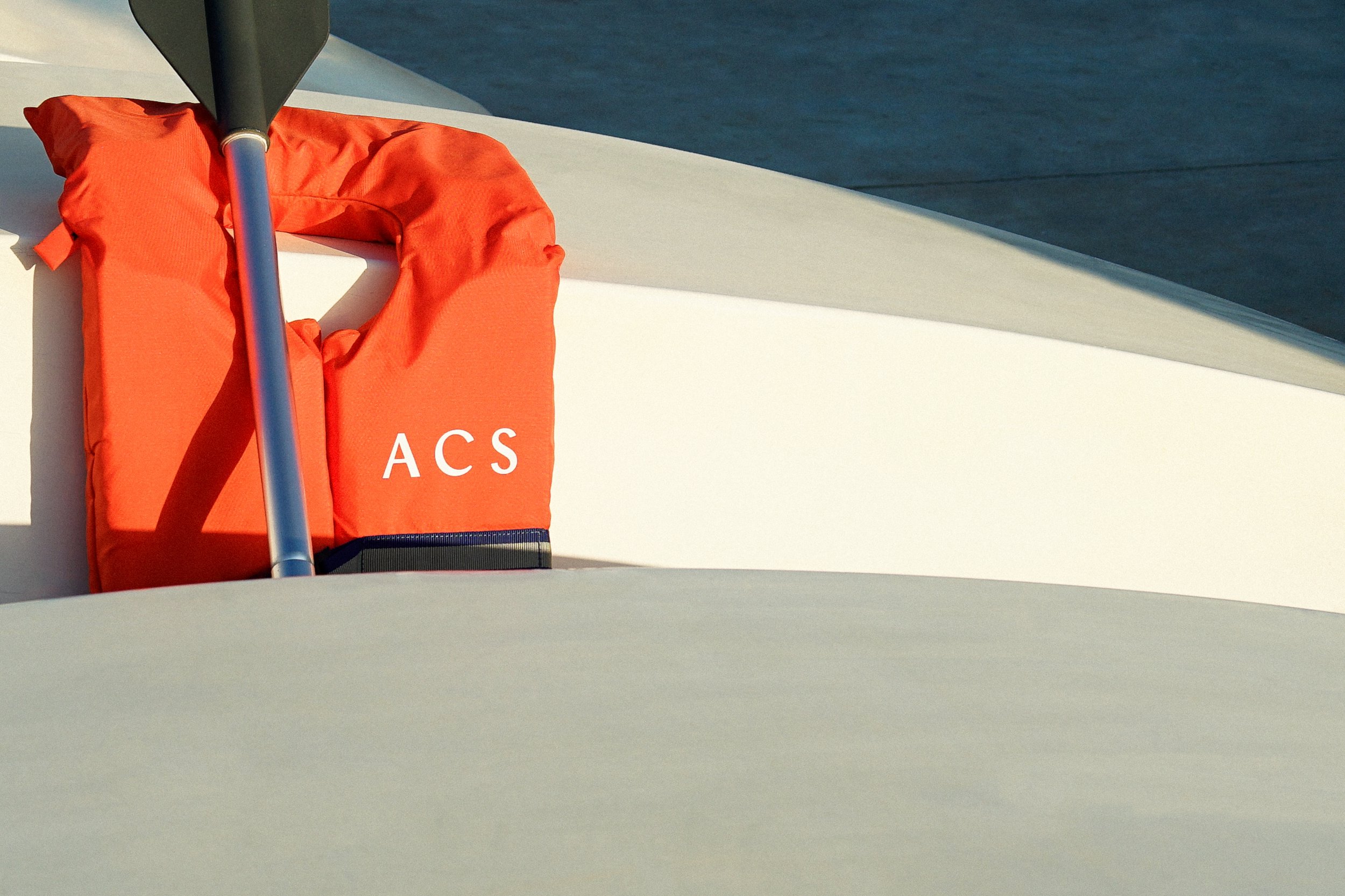
About
- Number of glyphs: 1250
- Number of weights: 7
- Number of languages: 116
- Available: desktop, web, social, app, epub
- Designer: Jan Horcik
- Date of release: 2021
- Version: 4.023
Character Set
- Basic Latin
- Latin-1 Supplement
- Latin Extended-A
- Latin Extended-B
- Combining Diacritical Marks
- Latin Extended Additional
- General Punctuation
- Currency Symbols
- Number Forms
- Enclosed Alphanumerics
- Dingbats
Features
- Access All Alternates
- Glyph Composition/Decomposition
- Localized Forms
- Subscript
- Scientific Inferiors
- Superscript
- Numerator
- Denominator
- Fractions
- Ordinals
- Contextual Alternates
- Lining Figures
- Proportional Figures
- Tabular Figures
- Oldstyle Figures
- Capitals to Small Caps
- Small Caps
- Case Sensitive Forms
- Discretionary Ligatures
- Slashed Zero
- Capital Spacing
- Stylistic Set 1
- Stylistic Set 2
- Stylistic Set 3
- Stylistic Set 4
- Stylistic Set 5
Languages
- Afrikaans
- Albanian
- Asu
- Basque
- Bemba
- Bena
- Bosnian
- Catalan
- Cebuano
- Chiga
- Colognian
- Cornish
- Corsican
- Croatian
- Czech
- Danish
- Dutch
- Embu
- English
- Estonian
- Faroese
- Filipino
- Finnish
- French
- Friulian
- Galician
- Ganda
- German
- Gusii
- Hungarian
- Icelandic
- Ido
- Inari Sami
- Indonesian
- Interlingua
- Irish
- Italian
- Javanese
- Jju
- Jola-Fonyi
- Kabuverdianu
- Kalaallisut
- Kalenjin
- Kamba
- Kikuyu
- Kinyarwanda
- Kurdish
- Latvian
- Lithuanian
- Lojban
- Low German
- Lower Sorbian
- Luo
- Luxembourgish
- Luyia
- Machame
- Makhuwa-Meetto
- Makonde
- Malagasy
- Malay
- Maltese
- Manx
- Maori
- Meru
- Morisyen
- North Ndebele
- Northern Sami
- Northern Sotho
- Norwegian Bokmål
- Norwegian Nynorsk
- Nyanja
- Nyankole
- Occitan
- Oromo
- Polish
- Portuguese
- Romanian
- Romansh
- Rombo
- Rundi
- Rwa
- Samburu
- Sango
- Sangu
- Sardinian
- Scottish Gaelic
- Sena
- Shambala
- Shona
- Slovak
- Slovenian
- Soga
- Somali
- South Ndebele
- Southern Sotho
- Spanish
- Swahili
- Swati
- Swedish
- Swiss German
- Taita
- Taroko
- Teso
- Tsonga
- Tswana
- Turkish
- Turkmen
- Upper Sorbian
- Vunjo
- Walloon
- Walser
- Welsh
- Western Frisian
- Wolof
- Xhosa
- Zulu
- Afrikaans
- Albanian
- Asu
- Basque
- Bemba
- Bena
- Bosnian
- Catalan
- Cebuano
- Chiga
- Colognian
- Cornish
- Corsican
- Croatian
- Czech
- Danish
- Dutch
- Embu
- English
- Estonian
- Faroese
- Filipino
- Finnish
- French
- Friulian
- Galician
- Ganda
- German
- Gusii
- Hungarian
- Icelandic
- Ido
- Inari Sami
- Indonesian
- Interlingua
- Irish
- Italian
- Javanese
- Jju
- Jola-Fonyi
- Kabuverdianu
- Kalaallisut
- Kalenjin
- Kamba
- Kikuyu
- Kinyarwanda
- Kurdish
- Latvian
- Lithuanian
- Lojban
- Low German
- Lower Sorbian
- Luo
- Luxembourgish
- Luyia
- Machame
- Makhuwa-Meetto
- Makonde
- Malagasy
- Malay
- Maltese
- Manx
- Maori
- Meru
- Morisyen
- North Ndebele
- Northern Sami
- Northern Sotho
- Norwegian Bokmål
- Norwegian Nynorsk
- Nyanja
- Nyankole
- Occitan
- Oromo
- Polish
- Portuguese
- Romanian
- Romansh
- Rombo
- Rundi
- Rwa
- Samburu
- Sango
- Sangu
- Sardinian
- Scottish Gaelic
- Sena
- Shambala
- Shona
- Slovak
- Slovenian
- Soga
- Somali
- South Ndebele
- Southern Sotho
- Spanish
- Swahili
- Swati
- Swedish
- Swiss German
- Taita
- Taroko
- Teso
- Tsonga
- Tswana
- Turkish
- Turkmen
- Upper Sorbian
- Vunjo
- Walloon
- Walser
- Welsh
- Western Frisian
- Wolof
- Xhosa
- Zulu
Select license from the tabs